Unveiling the Mechanics of Failure: How Mechanical Joints Succumb to the Forces of Time and Stress
3 min read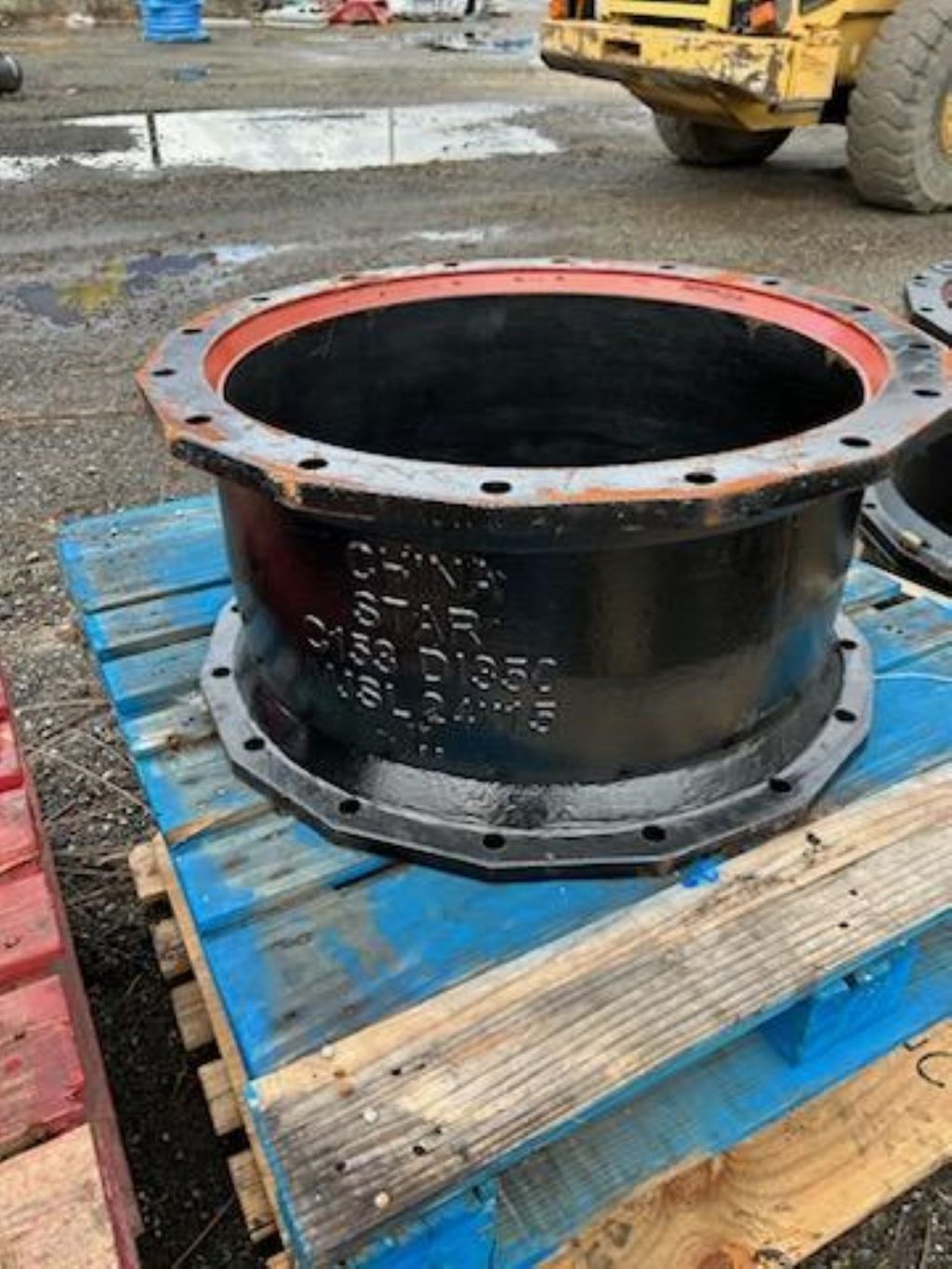
Mechanical joints serve as the critical links that hold various components together in engineering structures. However, the inevitable reality is that these joints are susceptible to failure over time, a phenomenon rooted in the intricate interplay of forces and materials. This exploration aims to dissect the mechanisms through which mechanical joints succumb to failure, providing insights into the complexities that engineers grapple with in designing resilient connections.
1. Understanding the Forces at Play:
At the core of mechanical joint failures are the forces to which they are subjected. Tensile, compressive, and shear forces, coupled with cyclic loading and vibrations, create an environment where joints may eventually yield. Understanding the magnitude and direction of these forces is fundamental to predicting and preventing failures.
2. Fatigue Failure:
One of the most prevalent modes of mechanical joint failure is fatigue. This occurs when a joint is subjected to repeated loading and unloading cycles, leading to microscopic cracks that gradually propagate until catastrophic failure occurs. Fatigue failures often evade detection until they reach a critical stage, emphasizing the need for proactive inspection and maintenance.
3. Brittle Fracture:
Brittle fracture is characterized by sudden and catastrophic failure without significant deformation. It often occurs in materials with low ductility, such as certain metals and ceramics. Identifying the factors that promote brittle fracture, such as low temperatures and high loading rates, is crucial for mitigating the risks associated with this mode of failure.
4. Corrosion-induced Failures:
Corrosion, a relentless force acting on joints exposed to harsh environments, can lead to material degradation and eventual failure. Understanding the corrosive agents at play, implementing corrosion-resistant materials, and employing protective coatings are strategies to combat this insidious form of joint deterioration.
5. Overloading and Yielding:
Exceeding the load-bearing capacity of a mechanical joint can result in overloading and yielding. This is particularly relevant in applications where safety margins are critical. Engineers must carefully consider the design specifications and factor in potential variations in loads to prevent overloading-induced failures.
6. Misalignments and Stress Concentrations:
Misalignments during assembly or operational conditions can introduce stress concentrations at specific points in a joint. These concentrations can accelerate fatigue and lead to premature failure. Minimizing misalignments through precise assembly and considering stress redistribution strategies are essential preventive measures.
7. Environmental Factors:
Environmental conditions, including temperature variations, humidity, and exposure to aggressive chemicals, can influence the rate of mechanical joint failures. Correlating the joint's material compatibility with the environmental conditions it will face is vital for predicting and preventing deterioration.
8. Advances in Joint Design and Materials:
The pursuit of failure-resistant joints has led to advances in joint design and the development of innovative materials. Incorporating features like stress relief zones, improved material properties, and smart materials that respond dynamically to changing conditions contributes to the resilience of modern mechanical joints.
Conclusion:
In the intricate dance between forces and materials, mechanical joints navigate a delicate balance that dictates their longevity and reliability. This exploration has peeled back the layers of how these joints succumb to failure, from the insidious progression of fatigue to the sudden impact of brittle fractures. As industries evolve, so too must the strategies employed by engineers to design joints that withstand the relentless forces of time and stress.